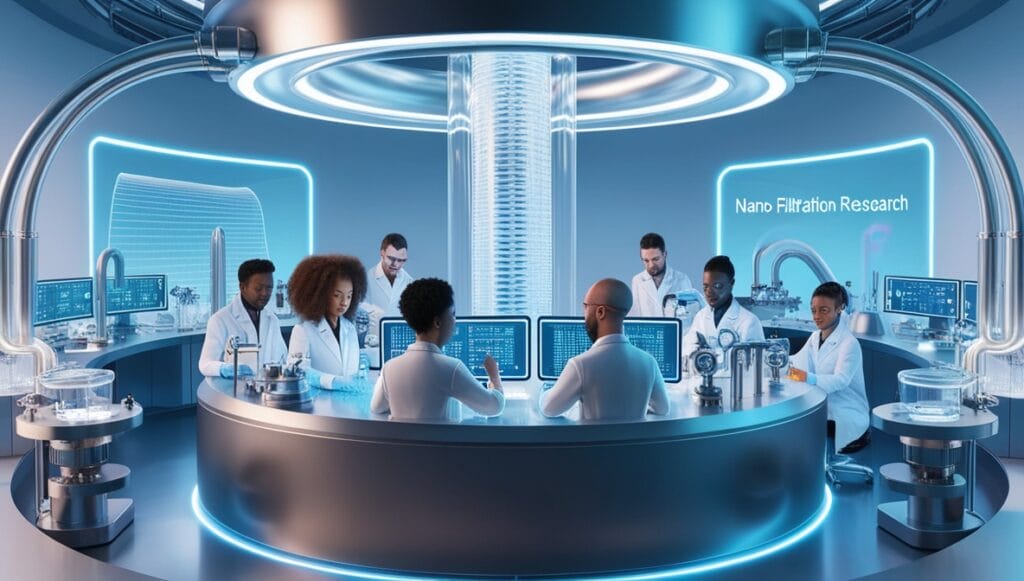
Membrane Technology and Research is a rapidly advancing field that plays a pivotal role in various industries, offering efficient solutions for separation processes such as filtration, purification, and desalination. This technology involves the use of semi-permeable membranes that selectively allow certain molecules to pass through while blocking others, making it ideal for applications in water treatment, gas separation, chemical processing, and biotechnology. Over the years, membrane technology has evolved significantly, with continuous research efforts leading to the development of innovative materials and techniques that enhance separation efficiency, reduce energy consumption, and lower operational costs.
One notable player in this sector is Membrane Technology and Research MTR Inc, a company renowned for its cutting-edge solutions and expertise in designing advanced membrane systems. MTR Inc. has made significant contributions to the industry by introducing high-performance membranes tailored for specific applications, such as carbon capture, natural gas processing, and industrial gas separation. Their technologies are not only transforming traditional separation methods but also paving the way for more sustainable and eco-friendly processes across various sectors.
Category | Details |
Scientific Processes | – Membrane technology involves the engineering approaches for constructing membranes that facilitate selective transport or rejection of substances. |
– Key processes include filtration, microfiltration, ultrafiltration, nanofiltration, and reverse osmosis. | |
Applications | – Water Treatment: Desalination, wastewater treatment, and drinking water production. |
– Chemical Processing: Separation of chemicals and solvents in various industries. | |
– Pharmaceuticals: Used in drug purification and production processes. | |
– Biotechnology: Involved in processes like protein purification and enzyme recovery. | |
– Food Industry: Concentration of beverages, recovery of proteins, and clarification of juices. | |
Function | – Facilitates the transport or rejection of substances between different mediums (e.g., liquid to gas). |
– Enables mechanical separation of gas and liquid streams, enhancing efficiency in various applications. | |
Characterization | – Involves determining key parameters such as pore size, functional groups, and material properties to ensure optimal performance in specific applications. |
– Characterization techniques include scanning electron microscopy (SEM), atomic force microscopy (AFM), and porometry to analyze membrane structure and performance. |
Definition:
Membrane technology is a versatile and rapidly growing field that involves the design, construction, and application of membranes for the selective transport or rejection of substances between different phases, typically in liquid or gas streams. These membranes act as semi-permeable barriers that allow certain molecules or ions to pass through while preventing others from crossing, based on factors such as size, charge, or chemical affinity. This selective separation process is essential in various industrial and environmental applications, offering a more efficient and energy-saving alternative to traditional separation methods.
The fundamental principle of membrane technology is based on the concept of selective permeability, where the membrane acts as a filter that separates components from a mixture. Depending on the type of membrane and the driving force applied—such as pressure, concentration, or electric potential—different substances can be separated or purified. This technology is widely employed in processes like water desalination, wastewater treatment, gas separation, food and beverage processing, and pharmaceutical manufacturing.
One of the most significant advantages of membrane technology is its ability to operate under mild conditions without the need for excessive heat or chemicals, making it a more sustainable and environmentally friendly solution. Moreover, advancements in membrane materials, such as polymeric, ceramic, and composite membranes, have expanded the range of applications and improved the efficiency and durability of these systems.
In recent years, companies like Membrane Technology and Research MTR Inc have been at the forefront of developing innovative membrane solutions for complex separation challenges. Their expertise and research efforts have led to the creation of advanced membranes that cater to specific industrial needs, such as carbon capture, hydrogen purification, and natural gas processing. This has solidified membrane technology as a critical tool in addressing global challenges related to water scarcity, energy efficiency, and environmental sustainability.
Applications of Membrane Technology
Membrane technology has emerged as a versatile and indispensable tool across various industries, owing to its efficiency, cost-effectiveness, and ability to provide high-purity separation processes. The technology is widely utilized in sectors such as water treatment, pharmaceuticals, biotechnology, food processing, and environmental protection, playing a crucial role in enhancing the quality and sustainability of products and processes.
1. Water Treatment
Water treatment is one of the most significant applications of membrane technology, where it is employed for processes like desalination, wastewater treatment, and purification of drinking water. Membrane-based processes such as reverse osmosis (RO), ultrafiltration (UF), nanofiltration (NF), and microfiltration (MF) are commonly used to remove contaminants, salts, bacteria, viruses, and other impurities from water sources. Reverse osmosis, for example, is extensively used for seawater desalination, transforming seawater into fresh, potable water. This application is particularly crucial in regions facing water scarcity, as it provides an efficient method to secure clean water supplies.
2. Pharmaceuticals
In the pharmaceutical industry, membrane technology is integral for processes such as drug purification, concentration, and separation. It ensures the removal of impurities, bacteria, and endotoxins from pharmaceutical products, guaranteeing that drugs meet stringent safety and quality standards. Membrane filtration is also used in the production of sterile water for injection and in processes like dialysis, where it helps in the selective removal of toxins from blood.
3. Biotechnology
The biotechnology sector utilizes membrane technology for the concentration, purification, and fractionation of biological molecules, including proteins, enzymes, and nucleic acids. Membranes play a crucial role in cell harvesting, fermentation processes, and the purification of bioproducts. Ultrafiltration and microfiltration membranes are particularly valuable in separating and concentrating biomolecules from complex mixtures, ensuring high purity and yield of the final products.
4. Food Processing
Membrane technology has revolutionized the food and beverage industry by providing efficient separation and purification processes that enhance product quality and safety. It is used for applications such as milk concentration, whey protein isolation, juice clarification, and the removal of impurities from wine and beer. For instance, ultrafiltration is widely employed in the dairy industry to concentrate milk proteins, while microfiltration helps in removing bacteria and spores, extending the shelf life of dairy products without compromising quality.
5. Environmental Protection
In the context of environmental protection, membrane technology offers sustainable solutions for treating industrial effluents, recovering valuable resources, and reducing pollution. It is used to remove heavy metals, organic pollutants, and hazardous chemicals from wastewater generated by industries such as mining, chemical processing, and textiles. Additionally, gas separation membranes are employed to capture greenhouse gases like carbon dioxide (CO₂) from industrial emissions, contributing to efforts to reduce carbon footprints and combat climate change.
Companies like Membrane Technology and Research MTR Inc have been instrumental in advancing membrane applications across these sectors, providing innovative solutions that address complex separation challenges. Their contributions have enhanced efficiency, sustainability, and environmental protection, making membrane technology a cornerstone of modern industrial processes.
Key Applications of Membrane Technology
Membrane technology has established itself as an essential tool across diverse fields, delivering efficient, cost-effective, and sustainable solutions for various processes. Some of the most significant applications include water treatment, energy production, and medical uses. Below is a detailed exploration of these key areas:
1. Water Treatment
Water treatment is one of the most established and extensive applications of membrane technology. Membrane processes such as reverse osmosis (RO), nanofiltration (NF), ultrafiltration (UF), and microfiltration (MF) are widely employed to purify water, making it suitable for drinking, industrial use, or safe environmental discharge.
- Drinking Water Production: Membrane technology plays a vital role in producing clean and safe drinking water. Reverse osmosis (RO) is the most commonly used process for desalination, where it effectively removes dissolved salts, minerals, and contaminants from seawater and brackish water. This technology is particularly beneficial for regions with limited access to fresh water, as it provides a reliable source of potable water.
- Wastewater Treatment: In wastewater treatment, membrane processes are used to remove contaminants, bacteria, viruses, suspended solids, and macromolecules from industrial and municipal wastewater. Ultrafiltration (UF) and microfiltration (MF) are often employed to separate and concentrate sludge, reduce turbidity, and ensure that the treated water meets environmental discharge standards. These processes contribute to water recycling and help in reducing the environmental impact of wastewater.
2. Energy Production
Membrane technology is increasingly being adopted in the energy sector, where it plays a crucial role in enhancing the efficiency of renewable energy systems and reducing environmental impacts.
- Fuel Cells: Membranes are integral components of fuel cells, which convert chemical energy from hydrogen into electricity. In proton exchange membrane fuel cells (PEMFCs), a proton exchange membrane acts as an electrolyte, allowing only protons to pass through while blocking electrons. This selective separation ensures the efficient generation of electricity and water as byproducts, making fuel cells a clean energy source.
- Biofuel Production: Membrane technology is also used in biofuel production to enhance the separation and purification processes. For example, membrane distillation and pervaporation are employed to separate water and impurities from bioethanol, resulting in higher purity levels. The use of mixed matrix membranes (MMMs), which incorporate nanoparticles into the polymer matrix, has further improved the efficiency and selectivity of biofuel production, leading to better yields and reduced energy consumption.
3. Medical Uses
Membrane technology has made significant contributions to the medical field, providing life-saving treatments and supporting various medical devices.
- Hemodialysis: One of the most well-known medical applications of membrane technology is in hemodialysis, a process used to treat patients with kidney failure. During hemodialysis, a semipermeable membrane acts as an artificial kidney, filtering out waste products, excess salts, and fluids from the blood. This process helps maintain the body’s electrolyte balance and remove toxins, ensuring that patients can lead healthier lives even with impaired kidney function.
- Oxygen Supply Systems: Membranes are also utilized in oxygen supply systems in medical settings, such as oxygen concentrators. These systems use gas separation membranes to extract oxygen from air, providing a continuous supply of purified oxygen to patients with respiratory conditions. This application is particularly critical for patients with chronic obstructive pulmonary disease (COPD) or those requiring supplemental oxygen therapy.
Companies like Membrane Technology and Research MTR Inc have been instrumental in advancing the applications of membranes in these sectors, developing high-performance membrane solutions that address the specific requirements of water treatment, energy production, and medical applications. Their innovative designs, such as mixed matrix membranes (MMMs), have significantly improved the efficiency, selectivity, and sustainability of these processes, making membrane technology an essential component in tackling some of the world’s most pressing challenges.
Types of Membrane Processes
Membrane processes are widely utilized for the separation and purification of substances across different industries, offering efficient and selective methods based on the nature and size of particles or molecules involved. These processes rely on different separation mechanisms, and recent advancements have introduced innovative techniques that further enhance their efficiency and application range. Below is a detailed look at the various types of membrane processes and innovative techniques in the field:
1. Separation Mechanisms
The choice of membrane process largely depends on the particle size, nature of the substance, and the desired separation outcome. The most commonly used membrane processes include:
- Microfiltration (MF): Microfiltration is a low-pressure membrane process used to separate particles in the range of 0.1 to 10 micrometers. It is effective in removing suspended solids, bacteria, and larger macromolecules from liquids, making it ideal for applications such as water purification, wastewater treatment, and the clarification of beverages like beer and wine. In this process, the membrane acts as a fine filter that allows water and dissolved salts to pass through while retaining larger particles.
- Ultrafiltration (UF): Ultrafiltration membranes have smaller pore sizes, typically ranging from 0.01 to 0.1 micrometers, and are used for separating proteins, colloids, and other macromolecules. It operates under moderate pressure and is widely applied in water treatment, dairy processing (e.g., whey protein concentration), and pharmaceutical manufacturing to ensure the removal of contaminants while retaining valuable components.
- Nanofiltration (NF): Nanofiltration is a medium-pressure process that lies between ultrafiltration and reverse osmosis in terms of selectivity. It can separate particles and molecules ranging from 0.001 to 0.01 micrometers in size, making it suitable for applications requiring selective removal of multivalent ions, dyes, organic compounds, and bacteria. Nanofiltration is commonly used for water softening, desalination of brackish water, and removing pesticides or organic pollutants from water sources.
- Reverse Osmosis (RO): Reverse osmosis is a high-pressure membrane process capable of removing ions, salts, and even the smallest dissolved molecules, with pore sizes less than 0.001 micrometers. This technique is widely used in desalination to produce fresh drinking water from seawater, as well as in industrial applications where high-purity water is required. In RO, water is forced through the membrane under high pressure, leaving behind salts and other impurities, resulting in highly purified water.
- Gas Separation: Gas separation membranes are used to separate gases based on differences in their permeability through the membrane material. These membranes are commonly applied in industries such as natural gas processing, hydrogen purification, and carbon capture. For example, in carbon capture applications, gas separation membranes selectively allow carbon dioxide (CO₂) to pass through, separating it from other gases in flue gas streams, which helps reduce greenhouse gas emissions.
2. Innovative Techniques
Recent advancements in membrane technology have led to the development of innovative techniques that enhance the efficiency, selectivity, and functionality of membrane processes. Some of these advancements include:
- Functionalized Membranes: Functionalized membranes have surface modifications that enable them to act as catalysts while simultaneously separating substances. These membranes are designed with specific chemical groups or nanoparticles that enhance their selectivity and reaction capabilities. For example, catalytic membranes can accelerate chemical reactions, such as the conversion of carbon dioxide (CO₂) into useful chemicals while separating it from gas streams, making the process more energy-efficient and sustainable.
- Mixed Matrix Membranes (MMMs): Mixed matrix membranes combine traditional polymer membranes with inorganic fillers, such as zeolites, metal-organic frameworks (MOFs), or carbon nanotubes, to improve separation performance. The incorporation of these fillers enhances the membrane’s selectivity, permeability, and thermal stability, making them highly effective for gas separation, pervaporation, and liquid separation processes. MMMs have found applications in the separation of challenging gas mixtures, such as natural gas purification and carbon capture.
- Membrane Distillation: Membrane distillation is a thermally driven process where a hydrophobic membrane separates a hot feed solution from a cooler permeate side. This technique is used to separate water from saline or contaminated solutions by taking advantage of the vapor pressure difference across the membrane. It is highly efficient in desalination, wastewater treatment, and concentration of heat-sensitive solutions, as it operates at lower temperatures compared to conventional distillation methods.
- Electrospun Nanofiber Membranes: Electrospun nanofiber membranes are made from ultrafine fibers with high surface area-to-volume ratios, resulting in enhanced permeability and selectivity. These membranes are gaining popularity in applications such as air filtration, water purification, and tissue engineering due to their exceptional filtration capabilities and mechanical strength. Their porous structure and ability to incorporate functional additives make them ideal for advanced separation and filtration processes.
Companies like Membrane Technology and Research MTR Inc have been at the forefront of developing these innovative membrane processes, contributing to advancements in efficiency, selectivity, and sustainability. By leveraging functionalized membranes and other cutting-edge techniques, they continue to expand the potential applications of membrane technology, addressing the growing demand for efficient separation processes across various industries.
Material Considerations in Membrane Technology
The choice of membrane materials is a critical factor in determining the efficiency, durability, and overall performance of membrane processes. The materials used must possess specific properties, such as chemical resistance, mechanical strength, thermal stability, and selectivity, to perform effectively in various applications. Below is a detailed exploration of common materials used in membrane construction and recent advancements aimed at enhancing membrane performance and sustainability.
1. Common Materials
Several synthetic polymers are commonly used to manufacture membranes due to their versatility, cost-effectiveness, and desirable properties. Some of the most widely used materials include:
- Polysulfone (PSF): Polysulfone is a popular membrane material known for its high thermal stability, chemical resistance, and mechanical strength. It is widely used in microfiltration (MF) and ultrafiltration (UF) membranes for water treatment, biotechnology, and food processing. Its excellent stability makes it suitable for handling harsh conditions, but polysulfone membranes are often hydrophobic, requiring surface modification to improve their wettability.
- Polyvinylidene Fluoride (PVDF): PVDF is another common material used in membrane manufacturing due to its outstanding chemical resistance, thermal stability, and hydrophobic nature. It is frequently employed in applications such as water treatment, wastewater recycling, and gas separation. PVDF membranes have a high degree of flexibility and mechanical strength, making them suitable for harsh industrial environments. Additionally, PVDF’s hydrophobicity can be modified to improve its affinity for water, making it more versatile.
- Polypropylene (PP): Polypropylene is a low-cost, chemically resistant, and thermally stable material used in microfiltration and ultrafiltration membranes. Its hydrophobic nature makes it suitable for applications in oil-water separation, gas separation, and the filtration of non-aqueous solutions. PP membranes are also commonly used in industrial wastewater treatment due to their durability and resistance to fouling.
- Cellulose Acetate: This material is commonly used for reverse osmosis (RO) membranes due to its relatively high flux and selectivity for water. It is an older but still effective material in applications like desalination and potable water production. However, cellulose acetate membranes are more sensitive to chlorine and high temperatures, requiring careful handling.
- Polyamide (PA): Polyamide is widely used in thin-film composite (TFC) membranes, especially for reverse osmosis and nanofiltration applications. TFC membranes consist of a polyamide layer coated on top of a support layer made from another polymer, such as polysulfone. The polyamide layer provides excellent selectivity and salt rejection capabilities, making it ideal for desalination, water purification, and industrial separation processes.
Biodegradable Alternatives
Given the growing environmental concerns regarding plastic waste, researchers are actively exploring the development of biodegradable and eco-friendly membrane materials. Some promising alternatives include:
- Polylactic Acid (PLA): PLA is a biodegradable polymer derived from renewable resources like corn starch or sugarcane. It has been investigated as a potential material for membrane production due to its biodegradability, low toxicity, and good mechanical properties. However, further research is needed to improve its chemical resistance and thermal stability for practical applications in filtration processes.
- Chitosan: Chitosan, derived from chitin (found in the shells of crustaceans), is another biodegradable material that has shown potential in membrane applications. It possesses natural antibacterial properties and can be used to create membranes for water purification, medical applications, and food processing. However, its mechanical strength and stability need enhancement for broader industrial use.
2. Performance Enhancements
To improve the efficiency, selectivity, and longevity of membranes, researchers have focused on integrating nanomaterials and advanced manufacturing techniques. These enhancements have shown great promise in addressing challenges such as fouling, mechanical stability, and separation performance:
- Integration of Nanomaterials: The incorporation of nanomaterials into membrane structures has emerged as a game-changing approach to enhancing membrane performance. Some commonly used nanomaterials include:
- Carbon Nanotubes (CNTs): Carbon nanotubes have unique properties such as high mechanical strength, electrical conductivity, and excellent permeability. When embedded in membrane structures, they create nanochannels that facilitate rapid and selective transport of water or gases, significantly improving flux rates and separation efficiency. CNT-enhanced membranes have been successfully applied in desalination, gas separation, and wastewater treatment.
- Graphene Oxide (GO): Graphene oxide has gained attention for its remarkable water permeability, mechanical strength, and antimicrobial properties. GO can be used to create highly selective and fouling-resistant membranes. For example, graphene oxide membranes have shown potential in improving the desalination process by providing high water flux while maintaining salt rejection.
- Metal-Organic Frameworks (MOFs): MOFs are porous materials with tunable pore sizes and high surface areas, making them excellent candidates for enhancing membrane selectivity. When integrated into polymeric membranes, MOFs can improve gas separation, water purification, and pervaporation processes by providing more efficient separation channels.
- Surface Modification: Surface modification techniques, such as plasma treatment, UV irradiation, and chemical grafting, are employed to improve the hydrophilicity, fouling resistance, and selectivity of membranes. These treatments alter the membrane surface properties, reducing the buildup of contaminants and improving overall performance. For instance, hydrophilic surface modifications can prevent fouling by making it more difficult for contaminants to adhere to the membrane.
- Electrospinning: Electrospinning is an advanced technique used to create nanofiber membranes with high porosity, large surface area, and excellent permeability. These membranes can be engineered to have specific pore sizes, making them suitable for applications such as air filtration, water treatment, and biomedical applications. The nanofiber structure enhances filtration efficiency and mechanical strength while maintaining a lightweight form.
Companies like Membrane Technology and Research MTR Inc have leveraged these material innovations to develop advanced membrane solutions with improved performance, durability, and efficiency. By integrating nanomaterials and employing surface modification techniques, they have successfully enhanced the capabilities of membranes, making them suitable for more demanding applications across various industries, including water treatment, energy production, and environmental protection.
Challenges and Future Directions in Membrane Technology
Despite its wide range of applications and growing adoption across various industries, membrane technology still faces challenges that need to be addressed to maximize its potential. Overcoming these challenges will pave the way for future advancements that can make membrane processes more efficient, cost-effective, and environmentally sustainable. Here’s a detailed examination of the key challenges and future directions in the field:
1. Economic Viability
The economic aspect of membrane technology is a significant factor affecting its adoption, especially in large-scale applications. Key challenges in this regard include:
- High Energy Consumption: Many membrane processes, particularly high-pressure techniques like reverse osmosis (RO) and nanofiltration (NF), require substantial amounts of energy to operate efficiently. This high energy demand can make the process expensive, especially for desalination or wastewater treatment applications where large volumes of water need to be processed. For instance, reverse osmosis desalination requires significant pressure to push water through the membrane, leading to elevated operational costs. Reducing energy consumption is a crucial aspect of making membrane technology more economically viable for widespread use.
- Fouling and Maintenance Costs: Fouling, the accumulation of unwanted materials (e.g., proteins, organic compounds, salts, and biofilms) on the membrane surface, is one of the most significant challenges that reduce membrane efficiency and lifespan. Fouling leads to decreased permeate flux and increased pressure requirements, resulting in higher energy consumption and maintenance costs. Frequent cleaning, replacement, or maintenance of membranes adds to the overall operational expenses, making the technology less attractive for cost-sensitive applications.
- Membrane Replacement and Waste Management: Membrane replacement is an ongoing cost in membrane-based systems, as membranes can become damaged or lose their functionality over time. Disposing of used or damaged membranes presents environmental and waste management challenges, particularly when dealing with non-biodegradable synthetic polymers. This disposal issue contributes to the overall life cycle cost of membrane systems.
2. Research Focus and Future Directions
Addressing these challenges requires a concerted effort in research and development to enhance the performance, efficiency, and sustainability of membrane processes. Key areas of research and future directions include:
- Development of Low-Energy Membrane Systems: Researchers are focusing on developing low-energy alternatives to conventional membrane processes. This includes designing membranes with higher permeability and selectivity, which can operate efficiently at lower pressures, thus reducing energy consumption. For example, the integration of nanomaterials like carbon nanotubes (CNTs) or graphene oxide (GO) into membrane structures can significantly enhance water flux while maintaining selectivity, leading to lower energy requirements.
- Anti-Fouling and Self-Cleaning Membranes: Developing membranes with anti-fouling properties is a crucial area of research. Surface modification techniques, such as coating membranes with hydrophilic or antibacterial agents, can help reduce fouling by preventing contaminants from adhering to the surface. Additionally, researchers are exploring self-cleaning membranes that use stimuli-responsive materials to release fouling agents when exposed to certain conditions, such as changes in temperature, pH, or light. These innovations can extend membrane life and reduce maintenance costs, making the technology more economically attractive.
- Exploration of New and Biodegradable Materials: As environmental sustainability becomes a priority, there is a growing interest in developing biodegradable and eco-friendly membrane materials. Biopolymers like polylactic acid (PLA) and chitosan, as well as other renewable materials, are being investigated as potential alternatives to conventional synthetic polymers. These materials offer the advantage of being more environmentally friendly and can help reduce the waste generated by used membranes. However, further research is needed to improve their durability, chemical resistance, and separation performance.
- Hybrid Membrane Systems: Combining membrane technology with other separation processes, such as adsorption, coagulation, or advanced oxidation, can enhance overall efficiency and address limitations associated with single-process systems. Hybrid membrane systems can offer improved selectivity, reduced fouling, and better energy efficiency. For example, combining membrane filtration with photocatalytic processes can result in membranes that not only separate contaminants but also degrade organic pollutants, providing a more comprehensive treatment solution.
- Mixed Matrix Membranes (MMMs) and Nanocomposite Membranes: Researchers are exploring the integration of inorganic fillers, such as zeolites, metal-organic frameworks (MOFs), or silica nanoparticles, into polymer membranes to create mixed matrix membranes (MMMs). These hybrid membranes exhibit enhanced permeability, selectivity, and thermal stability, making them suitable for more challenging applications, such as gas separation and solvent recovery. By leveraging the unique properties of these nanomaterials, MMMs can operate more efficiently under varying conditions, leading to broader industrial adoption.
- Smart Membranes with Adaptive Properties: Smart membranes that can adjust their permeability, selectivity, or functionality in response to external stimuli (e.g., temperature, pH, magnetic fields, or light) are an exciting area of research. These membranes can adapt to changing operating conditions, improving efficiency and reducing energy consumption. For instance, thermo-responsive membranes can alter their pore size when exposed to temperature changes, allowing for better control over separation processes.
Environmental Sustainability and Circular Economy
In addition to addressing economic and technical challenges, the future direction of membrane technology must also focus on environmental sustainability. This includes:
- Membrane Recycling and Reuse: Efforts are being made to develop recycling methods for used membranes, enabling their reuse in different applications or converting them into new products. For example, chemical or thermal treatments can recover valuable components from spent membranes, contributing to a circular economy approach.
- Green Manufacturing Processes: Adopting environmentally friendly manufacturing processes for membrane production, such as reducing solvent use, employing less energy-intensive methods, or using renewable raw materials, can help minimize the environmental footprint of membrane technology.
Companies like Membrane Technology and Research MTR Inc are actively engaged in addressing these challenges by developing advanced membrane solutions that focus on energy efficiency, durability, and environmental sustainability. By investing in research and development, they aim to create membrane systems that are not only economically viable but also aligned with sustainable practices, ensuring that membrane technology continues to evolve and meet the demands of modern industries in the years to come.
Conclusion:
Membrane technology stands as a dynamic and transformative field, evolving rapidly with advancements in materials science, nanotechnology, and engineering. Its diverse applications across sectors such as water purification, energy production, pharmaceuticals, and healthcare highlight its significance in addressing some of the most pressing challenges faced by modern society, including water scarcity, energy efficiency, and environmental sustainability.
As research continues to explore innovative materials, such as nanocomposites and biodegradable polymers, and incorporate cutting-edge processes like hybrid membrane systems and smart, adaptive membranes, the potential of membrane technology will expand even further. These advancements will not only enhance the efficiency and performance of membrane processes but also contribute to overcoming challenges related to energy consumption, fouling, and waste management.
Companies like Membrane Technology and Research MTR Inc are at the forefront of this evolution, driving innovation to make membrane technology more accessible, cost-effective, and environmentally sustainable. As this field continues to progress, membrane technology will undoubtedly play an increasingly vital role in shaping a sustainable future across various industries.
Faqs:
Q1: What are the latest advancements in membrane technology for water treatment?
Ans: Nanocomposite membranes and anti-fouling coatings have improved efficiency and durability.
Q2: How is membrane technology being used in biofuel production?
Ans: Membranes separate and purify biofuels, enhancing production efficiency and reducing energy consumption.
Q3: What are the benefits of using mixed matrix membranes in separation processes?
Ans: They offer higher selectivity, permeability, and thermal stability compared to conventional membranes.
Q4: How do functionalized membranes act as catalysts and filters simultaneously?
Ans: Functionalized membranes contain active sites that catalyze reactions while selectively filtering substances.
Q5: What role do membranes play in fuel cell technology?
Ans: Membranes act as electrolytes, allowing ion exchange while preventing fuel and oxidant crossover.